Ultrasound Contrast Agents
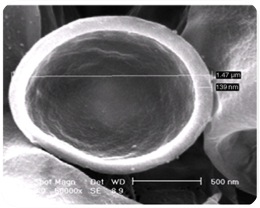
Ultrasound is a non-invasive medical imaging technique that relies on high-frequency sound waves to produce an image. Since most tissues are heterogeneous, a sound wave sent via a transducer acting as both a transmitter and a receiver will be scattered or reflected. It is this scattered energy, or backscatter, that returns to the transducer and from which an image can be produced. Over the past 10 years, ultrasound has gained increased usage due to technological advances and its ability to delivery real-time images. Some well-known uses of ultrasound include the in utero imaging of a fetus and the imaging of the heart to evaluate function. However, the applications and potential of ultrasound imaging extend well beyond pre-natal and cardiovascular imaging, including major human health issues such as tumor detection.
Ultrasound relies on a difference in properties at an interface between two different types of cells to produce an image. The difference in properties at the interface is referred to as an impedance mismatch. Impedance is related to the product of density and the speed of sound through a material. In a situation where there is a small impedance mismatch between two types of cells, such as between a tumor and a healthy breast, an ultrasound contrast agent is needed. A contrast agent provides a difference in impedance and makes imaging which was impossible now possible.
Angiogenesis is the formation of blood vessels from existing vessels and is an early sign in many types of developing tumors. However, angiogenesis is also involved in wound repair and inflammation. In the first step in angiogenesis, the parental vessels vasodilate and the basement membrane of the vessels begins to degrade via proteolytic enzymes. Endothelial cells begin to migrate into the extracellular space and proliferate to form a leading edge. The endothelial cells form tubules with lumen and begin to synthesize basement membrane. Finally, the tubules anastamose and recruit smooth muscle cells and pericytes to complete the vessel structure. Angiogenesis is directly linked to the growth and development of a tumor. Without sufficient nutrients, the tumor size cannot increase to greater than a few millimeters but with vasculature nearby, the tumor can become larger and eventually metastasize.
The start of angiogenesis occurs when a signaling molecule, such as an angiogenic growth factor, binds to the cell via a cell surface receptors and initiates a sequence, which results in new blood vessel formation. Integrins, a type of cell surface receptor, directly associate with the receptors that bind these growth factors and have the capacity to effect the outcome of the cell's behavior. The endothelial cells that line the blood vessels express receptors for angiogenic growth factors and during tumor development, may have enhanced expression of such receptors as a result of a lack of vasculature. As a tumor grows and develops, it requires vasculature and it is thought that chemotherapeutic agents bound to the growth factor receptors will result in localized cytotoxic effects. This observation suggests that if these receptors can be targeted, angiogenesis may be effected and thus, the growth of the developing tumor can be slowed down or even stopped.
With recent advances in cell and molecular biology as well as ultrasound imaging, research is being done to identify changes that occur in a pathologic condition using targeted imaging. Such imaging can be used to detect a change in tumor vasculature and the change with the malignancy of the tumor. This type of imaging requires that ultrasound contrast agents be modified with molecules, such as antibodies or peptides, which target the pathology, which can then be viewed using ultrasound imaging. In preliminary studies, such targeted contrast agents have been shown to be successful at targeting larger pathologies such as clots. Our studies show the possibility of coupling a marker and a contrast agent to create a targeted contrast agent, which can ultimately be used in the early detection, diagnosis, and treatment of a variety of pathologies.
Contrast Agent Research Activities:
- Development of contrast agent targeted to malignant cell lines
- Drug delivery systems using a contrast agent platform
- Nano-scale contrast agents
- Contrast agents for apoptosis signaling
Controlled Drug Delivery
Many drugs, such as vaccines, hormones and some antibiotics, require a delivery regime which is not zero order, for example vaccines need to be administered in pulses with time intervals between the pulses varying from a few weeks to months, while the hormone insulin needs to be delivered in response to elevated blood glucose levels. We approached this problem by combining two established technologies, liposomal entrapment of drug, and microencapsulation, to develop an entirely new modality, microencapsulated liposomes. This allows us two control points. No drug will be delivered until it is first released from the liposome, and once it is released the rate at which it is delivered to the body can be controlled by a rate controlling membrane on the microcapsule. The system also offers the advantage that the liposomes are protected from the immune system, and therefore are not taken up and delivered to the liver and spleen, and are also protected from phospholipid exchange that could occur if they were in the blood stream.
Liposomes are self assembling vesicles, in the nanometer to micron size range, composed of a bilayer of phospholipid molecules. The size and structure are determined by the method of preparation. When the liposomes form they entrap a portion of the aqueous environment, and if a drug, such as insulin, is dissolved in that aqueous medium then that will become entrapped.
We have chosen to make microcapsules by ionotropic gelation of the naturally occurring polymer, alginate. When an aqueous solution of sodium alginate is sprayed into calcium chloride solution, the drops gel upon contact with the calcium ions in the solution. If drug-containing liposomes are suspended in the sodium alginate before spraying, then they will become encapsulated. A rate-controlling membrane of a polycation such as poly-L-lysine can be coated on the microcapsules by simple contact with a polymer solution. This aqueous, mild microencapsulation method is ideal for encapsulation of sensitive structures such as liposomes. The size of the capsules can be controlled form about 50 µm to over 500 µm by the method of spraying.
As liposomes age they become leaky. We have developed a system of encapsulated liposomes, which will release their contents at different time points, depending on the composition and type of liposome. In this way, if a cocktail of liposomes is encapsulated, drug could be released in a series of pulses.
Management of diabetes requires delivery of exogenous insulin, preferably in response to elevated blood glucose levels. In the literature, we have found an amphipathic polymer, polyethyl acrylic acid (PEAA) that has been developed by Dr. D. Tirrell at the University of Massachusetts, which changes conformation and hydrophobicity with pH. At low pH the polymer adopts a globular hydrophobic conformation which interacts with the liposome bilayer causing it to become leaky. We have shown that the rate and extent of leakage can be controlled by the pH and the liposome to PEAA ratio. We are developing a responsive capsule which will contain liposomes loaded with insulin, PEAA and immobilized glucose oxidase enzyme(GOD). The scenario which we envision is that glucose will diffuse into the capsule, react at the GOD, the resulting gluconic acid will lower the pH, the PEAA will become protonated, change conformation and disrupt the bilayer, insulin will be released, pass out of the capsule, lower the glucose levels, the pH will re-equilibrate with the physiological pH of 7.4 and the leakage of insulin will cease.
Depending on the temperature, phospholipids in a membrane can be in either a gel or crystalline phase. The temperature at which they pass form one phase to another is called the phase, or glass transition temperature (Tm). Different phospholipids have different phase transition temperatures, and liposomes become leaky as they pass through the transition temperature of their constituent liposomes. We are investigating the use of temperature to induce leakage in encapsulated liposomes as a method of drug delivery to areas of elevated temperature such as tumors, inflammation and infection.
Research Activities:
- Development of pulsed and triggered release systems
- Liposomes
- Microcapsules
- Pulsed system for vaccines
- Triggered system for Insulin delivery
- Temperature sensitive liposomes for pulsed release
Ultrasound Triggered Drug Delivery
Medical ultrasound is a highly valuable diagnostic tool, especially when compared with other imaging modalities. It is non-invasive, real-time, portable, extremely safe compared to X-ray, and inexpensive relative to MRI. Recently, ultrasound contrast agents have been developed which when injected into the body, greatly enhance the contrast of the acquired image.
We have developed a method for producing hollow microcapsules, with diameters less then 10 microns so they can pass the pulmonary bed after intravenous injection. We describe the use of these agents for concomitant imaging and drug delivery. The drug-loaded contrast is imaged as it passes through the region of interest. At the same time drug release is triggered right at the insonated area. This study investigates two parameters: The effect of encapsulation method (solvent extraction and spray drying) on the drug payload by adsorption, and the effect of insonation parameters (such as time, insonation frequency and pressure) on the release profile. Hollow polylactide-co-glycolide microcapsules were prepared by the above techniques. The freeze-dried capsules were filtered and sized on a Horiba particle size analyzer. Zeta potential data was recorded pre- and post drug loading. FITC-BSA was used as a model high molecular weight bioactive drug. Release studies were performed in vitro in a release chamber fitted with an acoustic window. Insonation frequencies were in the medical range, at 5 and 10 MHz. Drug release was measured by an increase in FITC fluorescence in the release medium. The spray dried sample showed greater adsorption and drug release. The most dramatic increase in release was after 5 minutes for the 10 MHz low (1.25 MPa) pressure insonation, which showed 316% greater release than control, a total of 1.89 mg.
In order to reduce the size of the microcapsules, we have investigated a double emulsion method that produces capsules less then 3 microns.
Encapsulated Cells for Spinal Cord Regeneration
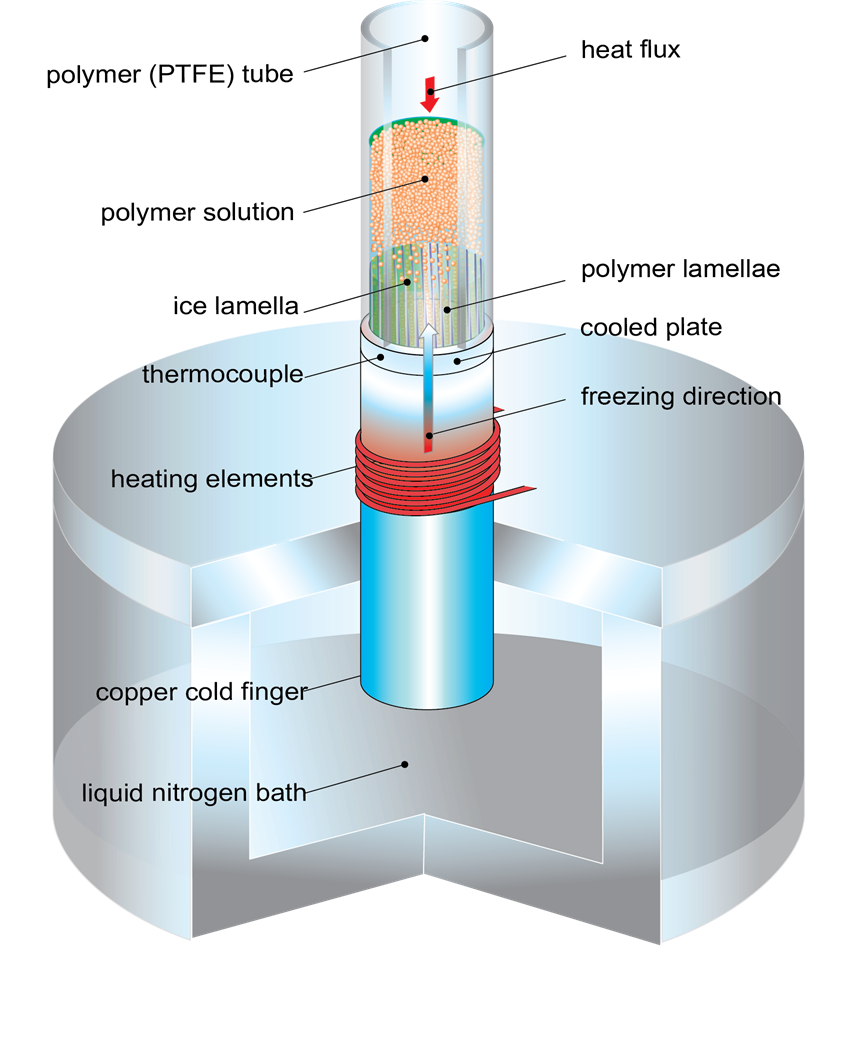
Approximately 10,000 people sustain new spinal cord injuries every year in the United States alone. The lack of effective treatment leads to a need for life long care and rehabilitation. The devastating effects of spinal cord injuries are due to (1) the death of neurons that cannot be replaced, (2) the failure of surviving neurons to regenerate their axons and (3) the inhospitable environment produced by the injury. Neurotrophic factors such as brain-derived neurotrophic factor (BDNF) can promote nerve regeneration and recovery of function. Transplantation of genetically engineered cells that produce these neurotrophic factors is one of the most promising approaches to treatment of spinal cord injuries and is expected to lead to development of protocols for clinical trials in the near future.
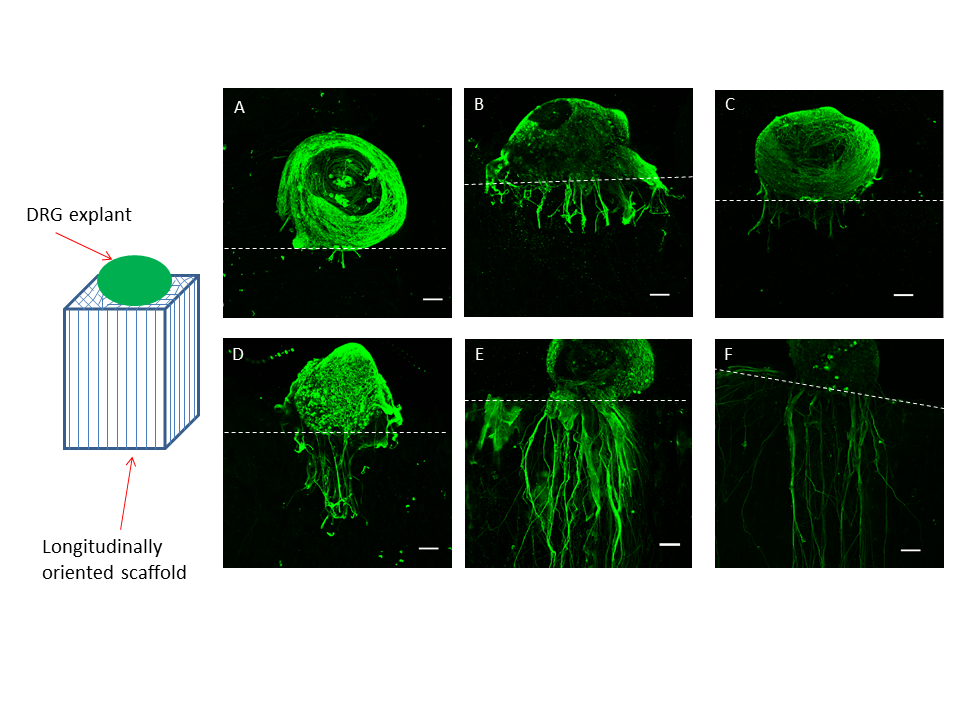
Ex vivo gene therapy is a strategy in which therapeutic genes are delivered to the host by a graft of genetically modified cells. Previously, we have shown that when primary fibroblasts are modified to produce BDNF and grafted into the injured spinal cord of adult rats, these cells survive, rescue axotomized neurons, promote regeneration and contribute to recovery of function (Liu et al., J. Neurosci. 1999 19:4370-4387). However, these grafted cells only survive in the injured rat spinal cord under strict immunosuppression protocols with Cyclosporin A. The disadvantages of immunosuppression are (1) the vulnerability of the recipient to infections, (2) potential development of tumors from the transplanted cells and (3) possible adverse effects on regeneration and recovery. Therefore, the efficacy of ex vivo grafting depends on the creation of an environment that supports the survival of the grafted cells and also acts as a permissive matrix for the regeneration of the neurons.
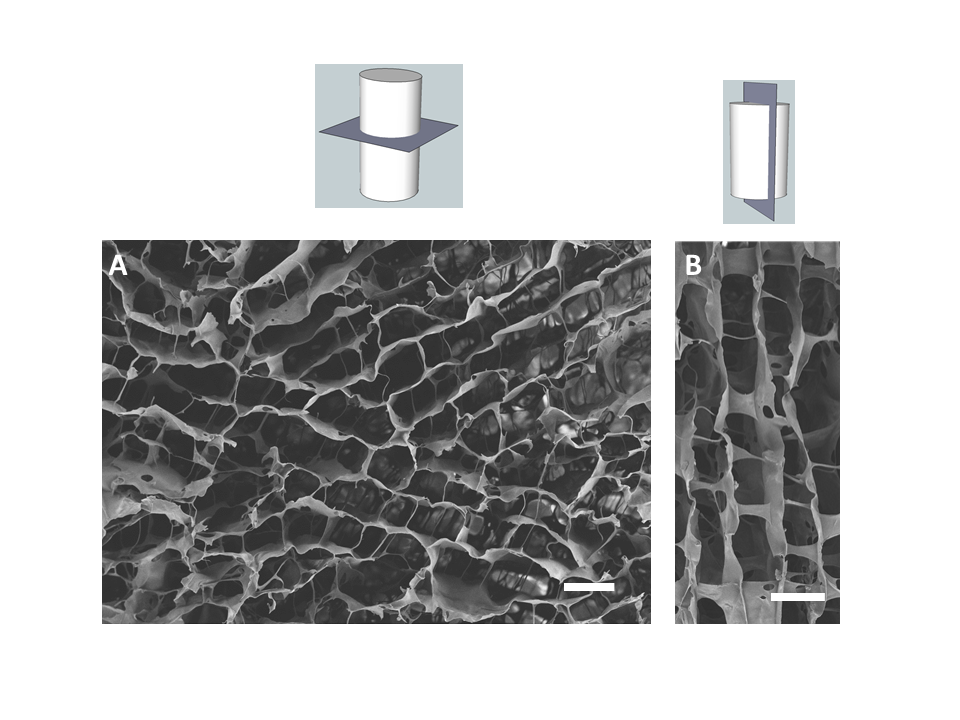
To address these problems, genetically modified cells can be encapsulated in a biocompatible polymer matrix that is semi-permeable, facilitating cell survival and allowing production and diffusion of the therapeutic product while protecting the cells from the host immune system. Alginate is a naturally occurring biocompatible linear polysaccharide consisting of alternating mannuronic and guluronic acid residues. The use of alginate for encapsulation of flavors, enzymes and cells such as pancreatic islets is well documented. Encapsulation of cells with alginate involves very mild conditions and non-toxic reagents. The surface of alginate capsules can easily be coated with a polypeptide such as poly-L-ornithine which provides a favorable surface for the growth of neurons. We examined (1) the important factors for encapsulation of BDNF-producing fibroblasts (BDNF/FB) with the biocompatible polysaccharide alginate, (2) the ability of the encapsulated cells to grow in vitro, and (3) the properties of the encapsulated cells following spinal cord grafting. We found that the encapsulated BDNF/FB proliferated and continued to express the transgene for at least 14 days in culture. In addition, when transplanted into a partial hemisected cervical adult rat spinal cord, without immune suppression, the encapsulated cells survived and appeared to provide a permissive environment for sprouting, as indicated by immunostaining for calcitonin gene-related peptide (CGRP), neurofilament, and microtubule associated protein 2 (MAP-2) after 14 days. We conclude that alginate encapsulation may provide an effective strategy for delivery of therapeutic products to the injured spinal cord in the absence of immune suppression.