Chapter 2.
Ceramic Hip Designs, Manufacturing, and Reliability
Steven M. Kurtz, PhD.
Jump to a Section
2.1 Introduction
2.2 Types of Contemporary Ceramic Bearing Designs.
2.3 Manufacturing of Ceramic Biomaterials
2.4 Reliability
2.5 Summary
2.6 Acknowledgements
2.7 References
2.1 Introduction
Contemporary ceramic hip implants embody both traditional and modern design elements as compared with their historical counterparts from the 1970s. This merging of time-tested design features, along with the progressive introduction of design innovations, has given rise to many distinctive ceramic implants described in the scientific literature [1, 2]. Currently the most well established design of ceramic components, both for femoral heads and acetabular liners, incorporates modular taper connections as discussed in Chapter 1. In 1990s, we have also seen the development of sandwich cup designs, as well as large diameter monoblock acetabular liner designs. We will discuss all three types of these contemporary designs in this chapter.
Along with component design, the materials used in ceramic implants have evolved over time, such that today the material of choice is increasingly zirconia toughened alumina nanocomposites. We briefly touched upon this material in Chapter 1, but here we devote some additional thought to how these nanocomposites are manufactured, including the choice of additives, and a crucial step, called proof testing, that is intended to improve the reliability of ceramic components.
There of course is a formal engineering definition of reliability, which embodies the concepts of survivorship and the successful functioning of a design or product. For the purposes of this chapter there are two key hurdles that modern ceramics have had to address in terms of improving their clinical success in the hands of surgeons. The first and most important of these challenges is the risk of brittle fracture; indeed this is such a crucial element of modern ceramics, starting in the 1970s, that fracture prevention is now integrated into the manufacturing process itself. Key processes that have been optimized in order to minimize clinical fractures include strict raw material selection; multiple thermal processing steps, including hot isostatic pressing; laser marking; and a final functional test of all manufactured components known as proof testing. More recently, over the past decade, bearing noise has emerged as a new topic related to ceramic implant reliability. While not related to failure of the implant per se, in certain rare cases the squeaking can be sufficiently loud as to require revision. We will cover both of these reliability topics in this chapter as well.
^ Return to Top
2.2 Types of Contemporary Ceramic Bearing Designs
Ceramic bearings in clinical use today include both ceramic articulating on a polyethylene liner, as well as ceramic-on-ceramic (COC) designs. The design principles for use in ceramic heads have been established for decades in terms of their taper locking geometry with the femoral stem. Over the years, perhaps the major design rules involving ceramic heads have involved an appreciation of impingement, which caused the failures of skirted heads of the early Autophor design from the 1980s [3], as well as the inherent limitations in the minimum diameter and lowest head offset options. The reader can find a detailed review describing the design considerations in modern ceramic heads [4], which in principle have remained well established since the 1970s. The latest generation of ceramic nanocomposites (e.g., BIOLOX®delta) has made it possible to extend the range of femoral head sizes and offsets beyond what was once possible using alumina (e.g., BIOLOX®forte), however the design details of the taper interlock design in these modern heads has not been universally adopted and no international standards exist defining the key design principles. For this reason it is the responsibility of the surgeon to ensure that the modular tapered components are compatible, especially during a revision procedure. The remainder of this section will focus on the changes in design of the acetabular liner, which has seen greater evolution than ceramic heads over the years.
Modern COC implants are modular or monoblock, and contemporary articulating surfaces are fabricated from alumina or, increasingly, ceramic nanocomposites. In certain cases, such as a revision scenario, a mixed combination of the two ceramic materials may be used (Figure 2.1). The acetabular insert may be fabricated entirely from alumina or nanocomposite, and fitted to the metal shell with a taper junction. Alternatively, the ceramic insert may be embedded within an UHMWPE liner. Currently, the majority of COC bearings are fabricated with a tapered insert design, rather than using the sandwich design [5], which today has currently fallen out of favor. Both types of COC designs are depicted in Figure 2.2.
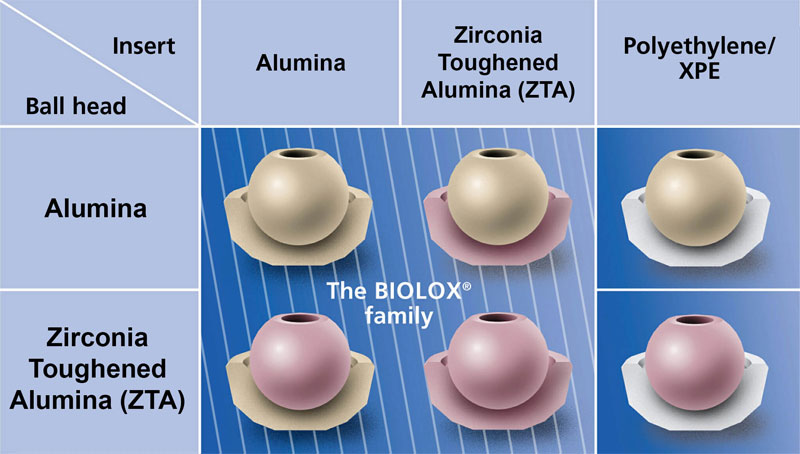
Figure 2.1.Contemporary ceramic bearing combinations including ceramic-on-polyethylene and ceramic-on-ceramic (provided courtesy of CeramTec AG, Plochingen, Germany).
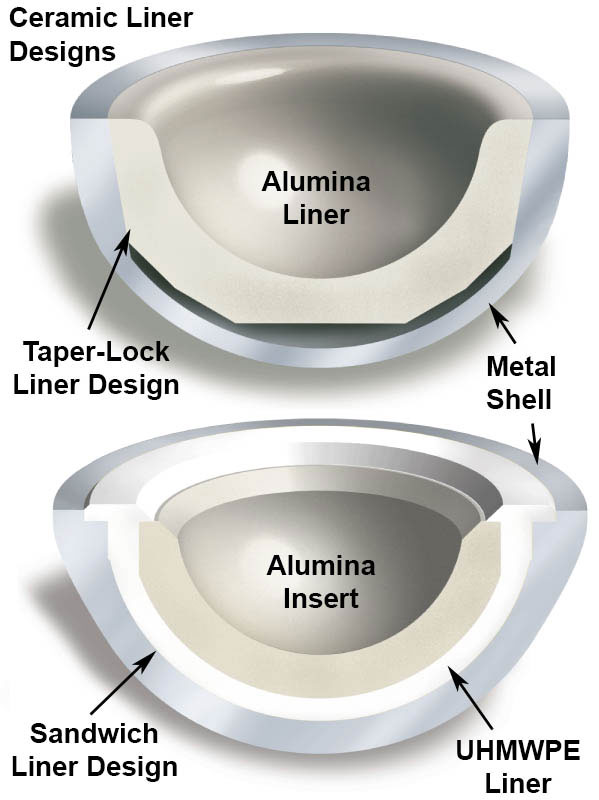
Figure 2.2.Two types of metal-backed acetabular component designs from the 1990s
2.2.1 Metal-Backed Taper Locking COC Designs
Metal-backed cup designs for COC bearings emerged in the 1990s as the main alternative to first-generation designs from the 1970s and 1980s, which were monoblock and fixed to bone either with cement or using a threaded interface. These early cemented and threaded COC designs were associated with elevated rates of aseptic loosening (exceeding 50% in some series [2]) and subsequently were abandoned during the 1990s in favor of metal-backed COC designs in which the cementless fixation to the bone was achieved via porous coating. There are many examples of early taper-locking COC designs (e.g., Plasmacup, Aesculap; Transcend, Wright Medical; ABG, Stryker) (Figure 2.3). Jeffers and Walter [2]have reviewed the clinical performance of these taper locking COC designs with clinical studies from the 1990s, which reported low rates of aseptic loosening (0-4.8%).
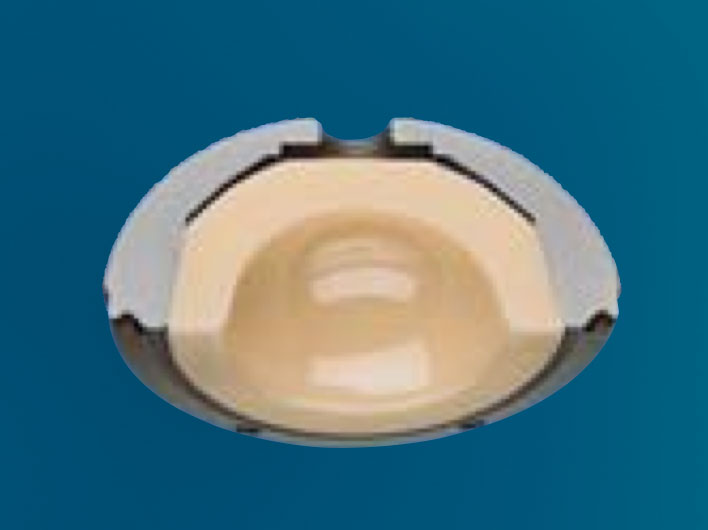
Figure 2.3.Example of a taper locking liner design (Plasmacup, Aesculap).
There are of course many proprietary variations of taper locking principles for ceramic liners across the industry, however they share the common feature of an equatorial conical taper in which the ceramic liner is locked into the metal shell (e.g., Figure 2.3). As an alternative to improve strength of alumina liners, for example, one orthopaedic manufacturer has devised a shrink fit, tapered titanium shell around the alumina liner as a variation on the modular taper design (Trident: Stryker Orthopedics, Mahwah, NJ) [6]. The tapered acetabular liner design is currently the dominant, most reliable technology for COC bearings in clinical use today. With over 20 years of successful clinical history documented in the scientific literature [2], the widespread use of COC bearings in orthopaedics can be attributed to taper locking designs.
Despite its success, the modular taper locking ceramic liner has certain inherent limitations that arise from its historical design principles. It is important to keep in mind that the locking mechanism was conceived for alumina ceramics at a time when the most commonly used head sizes for total hip replacement ranged between 22 and 32 mm. Over the past decade, with the development of higher strength zirconia toughened alumina nanocomposites (BIOLOX®delta), the thickness of tapered ceramic liners can be safely reduced to accommodate larger head sizes (e.g., 36-40 mm).
2.2.2 Metal-Backed Sandwich COC Designs
Modular metal backed sandwich designs emerged in the 1990s as an alternative to taper locking ceramic liner. The concept behind these liners was to maintain the modular interconnection between polyethylene and the metal shell, and replace the bearing surface with a hemispherical ceramic inlay (Figure 2.2). Clinical studies of sandwich designs were also reported in the literature starting in the 1990s with low rates of aseptic loosening, comparable to taper-locking designs [2].
Although the sandwich design was initially considered a viable design option for COC implants, these designs have since fallen out of favor due to a series of ceramic inlay fractures reported worldwide [7-14]. In the clinical experience of Kircher et al. in Germany, the fracture rate in a cohort of 50 sandwich-type ceramic liners was 18%, and was largely responsible for their overall fracture rate of 10% in their COC series [15]. A clinical trial in the United States was suspended due to high failure rates of the alumina insert in a COC sandwich design (14/315, 4.4%) [9]. The failure mechanism of this design was attributed to disruption of the ceramic-polyethylene interface, and fracture of the ceramic inlay was shown to occur after it became dislodged or displaced from the polyethylene liner. Sandwich-type COC designs have been reported with high fracture rates in clinical studies conducted in Europe [15], the United States [9], and in South Korea [10].
2.2.3 Large Diameter Ceramic Bearings/Resurfacing
Recently, a third type of COC design has been clinically introduced as a monoblock to provide increased joint stability (e.g., DELTAMOTION®: DePuy Synthes, Figure 2.4) [16]. Monoblock designs are preassembled by the manufacturer into the metal backing and are able to achieve a larger internal head diameter as compared to a traditional taper locking design for the same size of acetabulum. Modern monoblock COC designs also take advantage of the increased strength of zirconia toughened alumina nanocomposites to achieve thinner liners, and hence greater head diameters, than could be obtained using alumina. In addition to DELTAMOTION®, large diameter COC designs incorporating ZTA are also available from Zimmer Biomet (Maxera™) and Adler Ortho (AGILIS Ti-Por®). All three DELTAMOTION®, MaxeraTM and Agilis Ti-Por® designs employ BIOLOX®delta. Short term clinical data is still very limited.
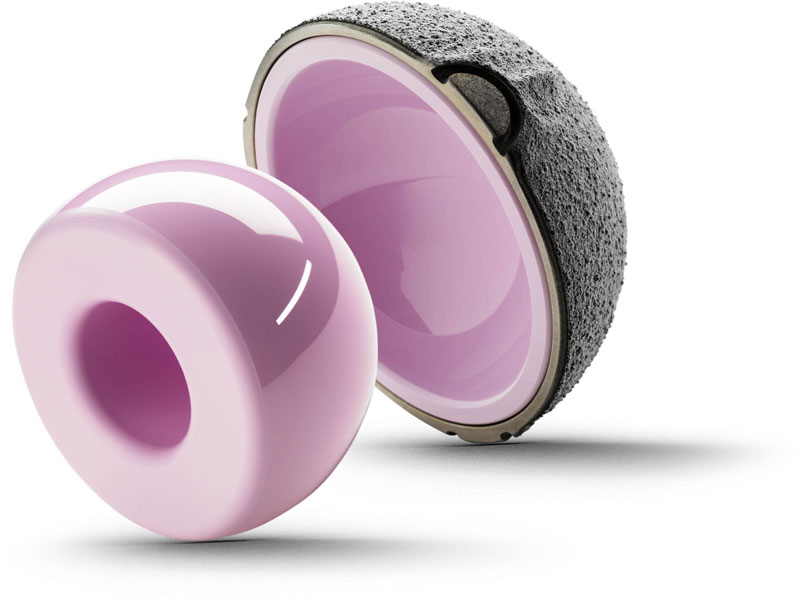
Figure 2.4.DELTAMOTION® large diameter total hip replacement, provided courtesy of DePuy Synthes (Warsaw, IN).
^ Return to Top
2.3 Manufacturing of Ceramic Biomaterials
The quality of ceramic implant components is defined by the strict selection of raw materials, processing parameters, and manufacturing conditions. Although the precise details of ceramic production remain proprietary, international efforts at standardization, increased medical device regulation and quality system requirements have all resulted in prescribed and controlled manufacturing processes for ceramic component suppliers to the orthopaedic industry. As we discussed in Chapter 1, a prominent manufacturer of ceramic components, i.e. St. Gobain Desmarquest, experienced elevated fracture rates following a change in their zirconia processing technology in 2001. As a result, St. Gobain issued a world-wide recall of their components, and then withdrew from the field of orthopaedics. Following the zirconia debacle, medical grade ceramics fell under increased regulatory around the world, especially in the US where COC bearings were under evaluation in regulated clinical trials by several manufacturers.
Today, there are certain enhanced manufacturing processes that are critical for producing reliable medical grade ceramic components [1, 17]. These include improved sintering techniques and hot isostatic pressing, laser marking, and proof testing. These are detailed further below. It is important to keep in mind the fact that these key developments are only a few in the list of continuous improvements made to ceramic components over the years.
Clean room processing
The precise control and cleanliness of the raw material used for medical ceramics is essential for minimizing the presence of contaminants. Ceramic impurities such as silicates, alkali oxides, iron oxides and others have the ability to create glassy phases in the grain boundaries in the microstructure of ceramics. In general, these glassy phases decrease corrosion resistance, mechanical strength, fatigue properties, and safety of the components. The voluntary international standards for these materials have been continuously updated to reflect the lower limits of these contaminants necessary to achieve the desired reliability.
Improved sintering and hot isostatic pressing
Over the years, the industry has learned that the process parameters for sintering temperature and time do not guarantee that density, average grain size, and ideal grain size distribution can be optimized at the same time. The application of multiple processes to achieve optimum values in all of these characteristics makes this achievable. For this reason, a lower sintering temperature is now used allowing for the optimization of grain size and its distribution. The use of a second process, hot isostatic pressing (HIP), is required to achieve an optimum density, as well as to minimize any internal voids or defects in the components. This process uses extremely high pressures (roughly 1,200 atmospheres) at a very high temperature (of more than 1,400°C, which is below the sintering temperature) in order to achieve full density of the ceramic materials. The last thermal process is a tempering cycle designed to reduce monoclinic zirconia phase content induced by mechanical processes.
Laser Marking
The marking of a ceramic component was historically done by engraving the component before sintering. Stress risers, when combined with the notch sensitivity and brittleness inherent in ceramics have always created a concern as to the surface effects created by these marking techniques on the service life of a ceramic component. It was therefore very appealing to apply laser marking technique to these components.
The application of the new laser marking technique has allowed manufacturers to reduce the effect of these marks on the surface. Laser marking allows for a more rounded, less deep and smoother profile on the markings. Nonetheless, laser marking is not a panacea. If it is not carried out correctly, it is possible to damage the surface and create imperfections that can affect the service life of the component. This process, laser marking, is an important element of the improvement in reliability of ceramic components.
Proof Testing
Another important development directed at improving the reliability of ceramic components has been the introduction of a non-destructive proof test developed and introduced into the production area by CeramTec in 1995 [18]. Before this test was introduced into the manufacturing process, ceramic components were subject to a finished product audit in which a statistical sampling plan was utilized and a certain number of components were destructively tested at the end of the production process. If these tests showed values within an accepted range, then the entire lot of components would be released for use. With the introduction of proof testing into the production cycle, 100% of the components manufactured can be subjected to this non-destructive test.
Although the details of proof testing are again confidential, commercial proof test protocols involve stressing the taper cone area, which is the critical area where the service stresses are expected to be the greatest and where most of the service fractures seem to originate in vivo [4]. The proof test described by Richter [18] is conducted by pressurizing the taper cone of a ball head with a fluid, which will result in tensile stresses on the inside surface of the cone, where service stresses are expected to be maximum. The value of the proof pressure is determined based on a variety of factors such as ball head size, neck length, and material. Components that survive the proof test are expected to withstand normal physiological loading. The test is designed to minimize the possibility of an internal defect affecting the reliability of a component. The proof testing of 100% of commercial components provides an invaluable assurance that ceramic components have sufficient reliability for in vivo service.
^ Return to Top
2.4 Reliability
2.1.1 Intraoperative Chipping and Component Fracture
One of the defining issues with ceramic components for hip replacement remains their in vivo fracture risk. A search of PubMed in July 2015 using the key words, “fracture” “ceramics,” and “hip” yielded 220 articles, with 17 of these papers published in 2014 and 5 of these papers published in the first half of 2015. Several of these papers documenting ceramic fracture are case reports, highlighting the unusual nature of these events. Regardless, ceramic fracture continues to be a clinically relevant topic for ceramic hip components used in the 21st century.
Fracture of ceramic taper locking acetabular components may occur during implantation if the liner is not properly aligned prior to impaction and seating within the metal shell. Intraoperative component fractures (“chipping”) were documented to occur in 2% to 3% of early cases performed in clinical trials of COC hips in the United States [6, 19]. These intraoperative fractures were produced by misalignment of the alumina liner in the metal shell, which results in a small fragment of the ceramic chipping off the rim when the insert is seated in the shell by impaction. Although rim chipping may be noticeable by the surgeon and corrected by substituting a new, undamaged liner prior to completing the procedure, the incidence of intraoperative chipping was sufficiently high to motivate one orthopaedic manufacturer to redesign the alumina liner in a protective titanium sleeve [6].
For modern ceramic heads, fabricated from alumina and ZTA, the risk of fracture is extremely low [20, 21]. Lee and Kim analyzed the reports of fracture reported to the manufacturer, CeramTec, for their femoral heads between 2000 and 2013 [20]. They found that the reported fracture incidence was 2.5/10,000 (0.0251%) for alumina (BIOLOX®forte) and 1/100,000 (0.001%) for ZTA (BIOLOX®delta). The 2014 report of the Australian Registry also contains data on the in vivo fracture of ceramic components [21]. The Australian Registry found that the clinical risk of revision for breakage for alumina femoral heads was 16 times greater than ZTA heads (Hazard Ratio 15.7, p = 0.007). They also reported that the reported fracture incidence was 6.48/10,000 (0.0648%) for alumina and 0.17/10,000 (0.0017%) for ZTA. The available data supports that, consistent with the in vitro fracture experiments and complaint data from CeramTec, ZTA exhibits significantly lower clinical fracture risk when compared with alumina.
The in vivo fracture of a ceramic component is a serious complication requiring immediate revision. The revision of a fractured femoral head may be complicated, because it typically fractures into multiple fragments, which may be difficult for the revising surgeon to completely clean from the surrounding tissues. There seems to be consensus that revision should consist of a complete synovectomy, followed by copious low pressure irrigation (small pieces generally float to the surface and can be extracted). Furthermore, the ceramic fragments typically damage the Morse taper of the femoral component. If the taper is severely damaged by in vivo abrasion from the ceramic fracture fragments, the entire stem must be revised. If the stem taper is largely undamaged a revision ceramic ball head (BIOLOX®Option) should be used. Due to the potential for trunnion damage, surgeons were once cautioned not to implant a new primary ceramic head during a revision if the stem is not also going to be revised [22]. Further discussion of revision options using ceramic heads will be covered in Chapter 4.
2.1.3 Bearing Noise
Total joint replacement systems are mechanical devices, and as such they may sometimes make noise as they work. The particular noise that began manifesting itself in ceramic total hip patients was a high-pitched noise or squeak. As a result, squeaking emerged as a new rare complication for hard-on-hard bearings in the mid to late 2000s after COC received FDA approval in the US. Although bearing noise has been documented in both MOM and COC bearings, the phenomenon has been studied in contemporary alumina-alumina bearings [23-32], as well as in COC bearings fabricated from ZTA [16, 33, 34]. The drop in the adoption of COC bearings in the United States between 2005 and 2007 is attributed to concerns by surgeons about bearing noise [35]. Since that time, a more mature understanding of this phenomenon has developed.
Although a formal classification system has not been established, bearing noise and squeaking is defined as a sound that originates from the artificial joint. Benign squeaking or bearing noise may be intermittent, low in intensity, or only associated with specific patient activities, such as bending down in a certain position [36]. Benign squeaking is tolerable to the patient and in some cases can be managed by physical rehabilitation, the use of orthotics, or by adjusting patient activities if the noise makes the patient embarrassed or otherwise uncomfortable [36]. Benign squeaking also does not appear to be associated with pain or with patient satisfaction of their THA [36], nor does it predict a clinical risk. However, in some severe cases, the noise is sufficiently loud and repetitive as to be considered unacceptable to the patient, necessitating revision. The literature, therefore, distinguishes between the incidence of squeaking, and the incidence of squeaking as a reason for revision [32].
Squeaking is thought to result from a breakdown of lubrication and high friction at the head-liner interface, producing a forced vibration of the entire arthroplasty construct [31, 37]. Analysis of retrieved components has documented edge loading wear and rim impingement in the majority of patients that were revised with complaints of squeaking [31]. On the other hand, squeaking has been observed in components with no evidence of edge loading wear and/or impingement upon retrieval. Edge loading is frequently noted in retrieved silent bearings [38]. Furthermore, the observation that squeaking is transient in some patients suggests that factors other than edge loading and impingement, such as metal debris entrapment, can also be responsible for acoustic emissions.
The prevalence of noise or squeaking in clinical series varies [24, 25, 36], with rates ranging from 2.7% to 25%, depending upon whether patients are surveyed directly, or surgeons are asked about the unsolicited complaints of noise from their patients. It is pertinent to mention that these different squeaking rates are directly related to the design of the metallic shell and stem. A recent meta-analysis estimated that the incidence of squeaking is about 4.2%, although the incidence of patients who were revised for squeaking was much lower at 0.2% [32]. As new large-diameter COC bearing designs, such as DELTAMOTION®, continue to be developed, clinical interest in this subject continues [16, 33, 34]. Many aspects about the prevalence, significance, and natural history of bearing noise have not yet been fully elucidated, especially for the newest COC designs.
^ Return to Top
2.5 Summary
In summary, this chapter was intended to provide the reader with an appreciation of the topics most relevant to the design, manufacture, and reliability of ceramic implants in clinical use today. These three subjects are interrelated. We will continue our discussion of reliability in the next chapter, in which we summarize the clinical performance of ceramic bearings in primary hip replacement.
2.6 Acknowledgements
The author would like to thank Kevin Ong, Exponent Inc., for editorial feedback on this chapter.
^ Return to Top
2.7 References
[1] Heros R, Pinconi C, Reinhardt C, Schneider N. Basic science of ceramics in total hip arthroplasty. The Adult Hip2015.
[2] Jeffers JR, Walter WL. Ceramic-on-ceramic bearings in hip arthroplasty: state of the art and the future. J Bone Joint Surg Br. 2012;94:735-45.
[3] Heisel J, Schmitt E. Implant fractures in ceramic hip endoprostheses. Z Orthop Ihre Grenzgeb. 1987;125:480-90.
[4] Huet R, Sakona A, Kurtz SM. Strength and reliability of alumina ceramic femoral heads: Review of design, testing, and retrieval analysis. J Mech Behav Biomed Mater. 2011;4:476-83.
[5] Willmann G. Fiction and facts concerning the reliability of ceramics in THR. In: Zippel H, Dietrich M, editors. Bioceramics in Joint Arthroplasty, 8th Biolox Symposium Proceedings. Darmstadt: Steinkopff Verlag; 2003. p. 193-6.
[6] D'Antonio J, Capello W, Manley M, Naughton M, Sutton K. Alumina ceramic bearings for total hip arthroplasty: five-year results of a prospective randomized study. Clin Orthop Relat Res. 2005:164-71.
[7] Kircher J, Bader R, Schroeder B, Mittelmeier W. Extremely high fracture rate of a modular acetabular component with a sandwich polyethylene ceramic insertion for THA: a preliminary report. Arch Orthop Trauma Surg. 2009;129:1145-50.
[8] Popescu D, Gallart X, Garcia S, Bori G, Tomas X, Riba J. Fracture of a ceramic liner in a total hip arthroplasty with a sandwich cup. Arch Orthop Trauma Surg. 2008;128:783-5.
[9] Poggie RA, Turgeon TR, Coutts RD. Failure analysis of a ceramic bearing acetabular component. J Bone Joint Surg Am. 2007;89:367-75.
[10] Ha YC, Kim SY, Kim HJ, Yoo JJ, Koo KH. Ceramic liner fracture after cementless alumina-on-alumina total hip arthroplasty. Clin Orthop Relat Res. 2007;458:106-10.
[11] Viste A, Chouteau J, Desmarchelier R, Fessy MH. Fractures of a sandwich ceramic liner at ten year follow-up. International orthopaedics. 2012;36:955-60.
[12] Lopes R, Philippeau JM, Passuti N, Gouin F. High rate of ceramic sandwich liner fracture. Clin Orthop Relat Res. 2012;470:1705-10.
[13] Szymanski C, Gueriot S, Boniface O, Deladerriere JY, Luneau S, Maynou C. Sandwich type ceramic liner fracture rate with the Atlas III socket: A study of 144 primary total hip replacements at a mean 74 months' follow-up. Orthop Traumatol Surg Res. 2011;97:494-500.
[14] Park YS, Park SJ, Lim SJ. Ten-year results after cementless THA with a sandwich-type alumina ceramic bearing. Orthopedics. 2010;33:796.
[15] Kircher J, Bader R, Schroeder B, Mittelmeier W. Extremely high fracture rate of a modular acetabular component with a sandwich polyethylene ceramic insertion for THA: a preliminary report. Arch Orthop Trauma Surg. 2008.
[16] Cai P, Hu Y, Xie J. Large-diameter Delta ceramic-on-ceramic versus common-sized ceramic-on-polyethylene bearings in THA. Orthopedics. 2012;35:e1307-13.
[17] Heros R, Willmann G. Ceramics In total hip arthroplasty: history, mechanical properties, clinical results, and current manufacturing state of the art. Semin Arthroplasty. 1998;9:114-22a.
[18] Richter HG, Willmann G. Reliability of ceramic components for total hip endoprostheses. Br Ceram Trans. 1999;98:29-34.
[19] Tateiwa T, Clarke IC, Williams PA, Garino J, Manaka M, Shishido T, Yamamoto K, Imakiire A. Ceramic total hip arthroplasty in the United States: safety and risk issues revisited. American journal of orthopedics (Belle Mead, NJ. 2008;37:E26-31.
[20] Lee GC, Kim RH. Reliability of ceramic heads in over 5.7 million hip replacements. Trans American Assoc Hip and Knee Surgeons (AAHKS). 2014:15.
[21] Australian Orthopaedic Association National Joint Replacement Registry. Annual Report. Adelaide: AOA; 2014.
[22] Krikler S, Schatzker J. Ceramic head failure. J Arthroplasty. 1995;10:860-2.
[23] Rosneck J, Klika A, Barsoum W. A rare complication of ceramic-on-ceramic bearings in total hip arthroplasty. J Arthroplasty. 2008;23:311-3.
[24] Restrepo C, Parvizi J, Kurtz SM, Sharkey PF, Hozack WJ, Rothman RH. The noisy ceramic hip: is component malpositioning the cause? J Arthroplasty. 2008;23:643-9.
[25] Baek SH, Kim SY. Cementless total hip arthroplasty with alumina bearings in patients younger than fifty with femoral head osteonecrosis. J Bone Joint Surg Am. 2008;90:1314-20.
[26] Yang CC, Kim RH, Dennis DA. The squeaking hip: a cause for concern-disagrees. Orthopedics. 2007;30:739-42.
[27] Walter WL, O'Toole G C, Walter WK, Ellis A, Zicat BA. Squeaking in ceramic-on-ceramic hips: the importance of acetabular component orientation. J Arthroplasty. 2007;22:496-503.
[28] Taylor S, Manley MT, Sutton K. The role of stripe wear in causing acoustic emissions from alumina ceramic-on-ceramic bearings. J Arthroplasty. 2007;22:47-51.
[29] Ranawat AS, Ranawat CS. The squeaking hip: a cause for concern-agrees. Orthopedics. 2007;30:738, 43.
[30] Eickmann TH, Clarke IC, Gustafson GA. Squeaking in a ceramic on ceramic total hip. In: Zippel H, Dietrich M, editors. Bioceramics in Joint Arthroplasty, 8th Biolox Symposium Proceedings. Darmstadt: Steinkopff Verlag; 2003. p. 187-91.
[31] Walter WL, Waters TS, Gillies M, Donohoo S, Kurtz SM, Ranawat AS, Hozack WJ, Tuke MA. Squeaking hips. J Bone Joint Surg Am. 2008;90 Suppl 4:102-11.
[32] Owen DH, Russell NC, Smith PN, Walter WL. An estimation of the incidence of squeaking and revision surgery for squeaking in ceramic-on-ceramic total hip replacement: a meta-analysis and report from the Australian Orthopaedic Association National Joint Registry. The bone & joint journal. 2014;96-B:181-7.
[33] Tai SM, Munir S, Walter WL, Pearce SJ, Walter WK, Zicat BA. Squeaking in Large Diameter Ceramic-on-Ceramic Bearings in Total Hip Arthroplasty. J Arthroplasty. 2015;30:282-5.
[34] McDonnell SM, Boyce G, Bare J, Young D, Shimmin AJ. The incidence of noise generation arising from the large-diameter Delta Motion ceramic total hip bearing. The bone & joint journal. 2013;95-B:160-5.
[35] Heros R. Personal communication. Ceramtec; 2008.
[36] Owen D, Russell N, Chia A, Thomas M. The natural history of ceramic-on-ceramic prosthetic hip squeak and its impact on patients. European journal of orthopaedic surgery & traumatology : orthopedie traumatologie. 2014;24:57-61.
[37] Hothan A, Lewerenz K, Weiss C, Hoffmann N, Morlock M, Huber G. Vibration transfer in the ball-stem contact interface of artificial hips. Med Eng Phys. 2013;35:1513-7.
[38] Walter WL, Insley GM, Walter WK, Tuke MA. Edge loading in third generation alumina ceramic-on-ceramic bearings: stripe wear. J Arthroplasty. 2004;19:402-13.